Leading scientists: energy crisis poses major 21st century threat, action needed now
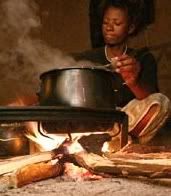
World leading energy experts from 15 academies of science have now analysed these pressing concerns and conclude in a major report that the energy crisis poses one of the greatest threats facing humanity this century. In 'Lighting the Way: Toward A Sustainable Energy Future', published by the InterAcademy Council, they highlight the perils of oil wars, of energy security, and of climate change driven by an addiction to fossil fuels. But in these dramatic challenges can be found the kernel of a major opportunity to enter a new era of sustainability, prosperity, social justice and security.
The InterAcademy Council has 15 members, including the national science academies of the United States, Britain, France, Germany, Brazil, China and India. The Council's report was authored by a 15-member panel whose co-chair was Nobel Physics laureate Steven Chu, a leading bioenergy researcher. It offers a consensus science roadmap published for directing global energy development and a scientific framework for securing economic growth and climate protection.
'Lighting the Way' calls for immediate and simultaneous action in three areas:
- Concerted efforts should be mounted to improve energy efficiency and reduce the carbon intensity of the world economy, including the worldwide introduction of price signals for carbon emissions
- Technologies should be developed and deployed for capturing and sequestering carbon from fossil fuels, particularly coal
- Development and deployment of renewable energy technologies should be accelerated in an environmentally responsible way
The poorest people on this planet should be supplied with basic, modern energy services.The report offers 9 key conclusions (see below), on the basis of which recommendations and calls for action are made with urgency to the world's governments, international institutions, science & technology and business communities, civil society and the public at large.
One of the conclusions is that biomass based renewable fuels and energy hold 'great promise for simultaneously addressing climate-change and energy-security concerns.' The report also calls for the introduction of carbon-negative bioenergy, by coupling carbon capture and storage technologies to biomass combustion and co-firing. Non-biomass renewables like wind and solar face some major challenges, but can seriously contribute to bringing modern energy to developing countries. The report further calls, with caution, for more investments in next-generation nuclear facilities. Other dawning technologies, such as plug-in hybrid cars and hydrogen fuel cells for energy storage, can make an important niche contribution.
The 'business as usual' energy path we are on today is not sustainable and is counter to the long-term prosperity of every nation. This report stresses the urgency of the energy problem, and then goes on to describe technologies that can be applied today, needed scientific and technological innovations, and policy tools that could be used to help policy makers guide their countries toward a more prosperous, secure and environmentally sound energy future. - Nobel Laureate Steven Chu, co-chair, director of the Lawrence Berkeley National LabHumankind has faced daunting problems in every age, but today’s generation confronts a unique set of challenges. The environmental systems on which all life depends are being threatened locally, regionally, and at a planetary level by human actions. And even as great numbers of people enjoy unprecedented levels of material prosperity, a greater number remains mired in chronic poverty, without access to the most basic of modern services and amenities and with minimal opportunities for social (e.g., educational) and economic advancement. At the same time, instability and conflict in many parts of the world have created profound new security risks:

Energy is critical to human development and connects in fundamental ways to all of these challenges. As a result, the transition to sustainable energy resources and systems provides an opportunity to address multiple environmental, economic, and development needs. From an environmental perspective, it is becoming increasingly clear that humanity’s current energy habits must change to reduce significant public health risks, avoid placing intolerable stresses on critical natural systems, and, in particular, to manage the substantial risks posed by global climate change.
By spurring the development of alternatives to today’s conventional fuels, a sustainable energy transition could also help to address the energy security concerns that are again at the forefront of many nations’ domestic and foreign policy agendas, thereby reducing the likelihood that competition for finite and unevenly distributed oil and gas resources will fuel growing geopolitical tensions in the decades ahead. Finally, increased access to clean, affordable, high-quality fuels and electricity could generate multiple benefits for the world’s poor, easing the day-to-day struggle to secure basic means of survival; enhancing educational opportunities; reducing substantial pollution-related health risks; freeing up scarce capital and human resources; facilitating the delivery of essential services, including basic medical care; and mitigating local environmental degradation.
Energy, in short, is central to the challenge of sustainability in all its dimensions: social, economic, and environmental. To this generation falls the task of charting a new course. Now and in the decades ahead no policy objective is more urgent than that of finding ways to produce and use energy that limit environmental degradation, preserve the integrity of underlying natural systems, and support rather than undermine progress toward a more stable, peaceful, equitable, and humane world. Many of the insights, knowledge, and tools needed to accomplish this transition already exist but more will almost certainly be needed. At bottom the decisive question comes down to this: Can we humans collectively grasp the magnitude of the problem and muster the leadership, endurance, and will to get the job done?
The enormity of the challenge
The task is as daunting as it is complex. Its dimensions are at once social, technological, economic, and political. They are also global. People everywhere around the world play a role in shaping the energy future through their behavior, lifestyle choices, and preferences. And all share a significant stake in achieving sustainable outcomes.
The momentum behind current energy trends is enormous and will be difficult to check in the context of high levels of existing consumption in many industrialized countries; continued population growth; rapid industrialization in developing countries; an entrenched, capital-intensive and long-lived energy infrastructure; and rising demand for energy-related services and amenities around the world. Although wide disparities exist in per capita energy consumption at the country level, relatively wealthy households everywhere tend to acquire similar energy-using devices. Therefore, the challenge and the opportunity exists—in industrialized and developing countries alike—to address resulting energy needs in a sustainable manner through effective demand- and supply-side solutions.
The prospects for success depend to a significant extent on whether nations can work together to ensure that the necessary financial resources, technical expertise, and political will are directed to accelerating the deployment of cleaner and more efficient technologies in the world’s rapidly industrializing economies. At the same time, current inequities that leave a large portion of the world’s population without access to modern forms of energy and therefore deprived of basic opportunities for human and economic development must also be addressed.
This could be achieved without compromising other sustainability objectives, particularly if simultaneous progress is achieved toward introducing new technologies and reducing energy intensity elsewhere throughout the world economy. The process of shifting away from a business-as-usual trajectory will necessarily be gradual and iterative: because essential elements of the energy infrastructure have an expected life on the order of one to several decades, dramatic changes in the macroscopic energy landscape will take time. The inevitable lag in the system, however, also creates grounds for great urgency. In light of growing environmental and energy security risks, significant global efforts to transit to a different landscape must begin within the next ten years. Delay only increases the difficulty of managing problems created by the world’s current energy systems, as well as the likelihood that more disruptive and costly adjustments will need to be made later.

In light of the widely held expectation that relatively cheap and readily accessible reserves of conventional petroleum will peak over the next few decades and mounting evidence that the responsible mitigation of climate-change risks will require significant reductions in global greenhouse gas emissions within the same timeframe, the scale of the mismatch between today’s energy trends and tomorrow’s sustainability needs speaks for itself.
Energy demand and efficiency
Achieving sustainability objectives will require changes not only in the way energy is supplied, but in the way it is used. Reducing the amount of energy required to deliver various goods, services, or amenities is one way to address the negative externalities associated with current energy systems and provides an essential complement to efforts aimed at changing the mix of energy supply technologies and resources.
Opportunities for improvement on the demand side of the energy equation are as rich and diverse as those on the supply side, and frequently offer significant near-term and long-term economic benefits. Widely varying per capita or per gross domestic product (GDP) levels of energy consumption across countries with comparable living standards—though certainly partly attributable to geographic, structural, and other factors—suggest that the potential to reduce energy consumption in many countries is substantial and can be achieved while simultaneously achieving significant quality-of-life improvements for the world’s poorest citizens.

In most countries, energy intensity — that is, the ratio of energy consumed to goods and services provided — has been declining, albeit not at a rate sufficient to offset overall economic growth and reduce energy consumption in absolute terms. Boosting this rate of intensity decline should be a broadly held, public policy priority.

Experience points to the availability of policy instruments for overcoming barriers to investments in improved efficiency even when such investments, based on energy and cost considerations alone, are highly cost-effective. The improvements in refrigerator technology that occurred as a result of appliance efficiency standards in the United States provide a compelling example of how public policy intervention can spur innovation, making it possible to achieve substantial efficiency gains while maintaining or improving the quality of the product or service being provided. Other examples can be found in efficiency standards for buildings, vehicles, and equipment; in addition to information and technical programs and financial incentive mechanisms.
Energy Supply

Energy security concerns — particularly related to the availability of relatively cheap, conventional supplies of petroleum and, to a lesser extent, of natural gas — continue to be important drivers of national energy policy in many countries and a potent source of ongoing geopolitical tensions and economic vulnerability. Nevertheless, environmental limits, rather than supply constraints, seem likely to emerge as the more fundamental challenge associated with continued reliance on fossil fuels. World coal reserves alone are adequate to fuel several centuries of continued consumption at current levels and could provide a source of petroleum alternatives in the future. Without some means of addressing carbon emissions, however, continued reliance on coal for a large share of the world’s future energy mix would pose unacceptable climate-change risks.
Achieving sustainability objectives will require significant shifts in the current mix of supply resources toward a much larger role for low-carbon technologies and renewable energy sources, including advanced biofuels. The planet’s untapped renewable energy potential, in particular, is enormous and widely distributed in industrialized and developing countries alike. In many settings, exploiting this potential offers unique opportunities to advance both environmental and economic development objectives.
Recent developments, including substantial policy commitments, dramatic cost declines, and strong growth in many renewable energy industries are promising. However, significant technological and market hurdles remain and must be overcome for renewable energy to play a significantly larger role in the world’s energy mix. Advances in energy storage and conversion technologies and in enhancing long-distance electric transmission capability could do much to expand the resource base and reduce the costs associated with renewable energy development. Meanwhile, it is important to note that recent substantial growth in installed renewable capacity worldwide has been largely driven by the introduction of aggressive policies and incentives in a handful of countries. The expansion of similar commitments to other countries would further accelerate current rates of deployment and spur additional investment in continued technology improvements.

Integrated bio-refineries could, in the future, allow for the efficient co-production of electric power, liquid fuels, and other valuable co-products from sustainably managed biomass resources. Greatly expanded reliance on biofuels will, however, require further progress in reducing production costs; minimizing land, water, and fertilizer use; and addressing potential impacts on biodiversity. Biofuels options based on the conversion of lignocellulose rather than starches appear more promising in terms of minimizing competition between growing food and producing energy and in terms of maximizing the environmental benefits associated with biomass-based transportation fuels.
It will be equally important to hasten the development and deployment of a less carbon-intensive mix of fossil fuel-based technologies. Natural gas, in particular, has a critical role to play as a bridge fuel in the transition to more sustainable energy systems. Assuring access to adequate supplies of this relatively clean resource and promoting the diffusion of efficient gas technologies in a variety of applications is therefore an important public policy priority for the near to medium term.
Simultaneously, great urgency must be given to developing and commercializing technologies that would allow for the continued use of coal—the world’s most abundant fossil-fuel resource—in a manner that does not pose intolerable environmental risks. Despite increased scientific certainty and growing concern about climate change, the construction of long-lived, conventional, coal-fired power plants has continued and even accelerated in recent years. The substantial expansion of coal capacity that is now underway around the world may pose the single greatest challenge to future efforts aimed at stabilizing carbon dioxide levels in the atmosphere. Managing the greenhouse gas ‘footprint’ of this existing capital stock, while making the transition to advanced conversion technologies that incorporate carbon capture and storage, thus represents a critical technological and economic challenge.
Nuclear technology could continue to contribute to future low-carbon energy supplies, provided significant concerns in terms of weapons proliferation, waste disposal, cost, and public safety (including vulnerability to acts of terrorism) can be—and are—addressed.
The role of government and the contribution of science and technology

Where the political will exists to create the conditions for a sustainable energy transition, a wide variety of policy instruments are available, from market incentives such as a price or cap on carbon emissions (which can be especially effective in influencing long-term capital investment decisions) to efficiency standards and building codes, which may be more effective than price signals in bringing about change on the end-use side of the equation. Longer term, important policy opportunities also exist at the level of city and land-use planning, including improved delivery systems for energy, water, and other services, as well as advanced mobility systems.
Science and technology (S&T) clearly have a major role to play in maximizing the potential and reducing the cost of existing energy options while also developing new technologies that will expand the menu of future options (table shows major research opportunities in the energy sector, click to enlarge). To make good on this promise, the S&T community must have access to the resources needed to pursue already promising research areas and to explore more distant possibilities. Current worldwide investment in energy research and development is widely considered to be inadequate to the challenges at hand.
Accordingly, a substantial increase—on the order of at least a doubling of current expenditures—in the public and private resources directed to advancing critical energy technology priorities is needed. Cutting subsidies to established energy industries could provide some of the resources needed while simultaneously reducing incentives for excess consumption and other distortions that remain common to energy markets in many parts of the world. It will be necessary to ensure that public expenditures in the future are directed and applied more effectively, both to address well-defined priorities and targets for research and development in critical energy technologies and to pursue needed advances in basic science. At the same time, it will be important to enhance collaboration, cooperation, and coordination across institutions and national boundaries in the effort to deploy improved technologies.
Achieving a sustainable energy future requires the participation of all. But there is a division of labor in implementing the various recommendations of this report. The Study Panel has identified the following principal ‘actors’ that must take responsibility for achieving results:
- Multi-national organizations (e.g., United Nations, World Bank, regional development banks)
- Governments (national, regional, and local)
- S&T community (and academia)
- Private sector (businesses, industry, foundations)
- Nongovernmental organizations (NGOs)
- Media
- General public
Conclusions, recommendations, actions
Based on the key points developed in this report, the Study Panel offers the following conclusions with recommendations and respective actions by the principal actors.
Conclusion 1: the poor first
Meeting the basic energy needs of the poorest people on this planet is a moral and social imperative that can and must be pursued in concert with sustainability objectives. Today, an estimated 2.4 billion people use coal, charcoal, firewood, agricultural residues, or dung as their primary cooking fuel. Roughly 1.6 billion people worldwide live without electricity. Vast numbers of people, especially women and girls, are deprived of economic and educational opportunities without access to affordable, basic labor-saving devices or adequate lighting, added to the time each day spent gathering fuel and water.
The indoor air pollution caused by traditional cooking fuels exposes millions of families to substantial health risks. Providing modern forms of energy to the world’s poor could generate multiple benefits, easing the day-to-day struggle to secure basic means of survival; reducing substantial pollution-related health risks; freeing up scarce capital and human resources; facilitating the delivery of essential services, including basic medical care; and mitigating local environmental degradation. Receiving increased international attention, these linkages were a major focus of the 2002 World Summit for Sustainable Development in Johannesburg, which recognized the importance of expanded access to reliable and affordable energy services as a prerequisite for achieving the United Nation’s Millennium Development Goals.
RECOMMENDATIONS
Place priority on achieving much greater access of the world's poor to clean, affordable, high-quality fuels and electricity. The challenge of expanding access to modern forms of energy revolves primarily around issues of social equity and distribution — the fundamental problem is not one of inadequate global resources, unacceptable environmental damage, or unavailable technologies. Addressing the basic energy needs of the world’s poor is clearly central to the larger goal of sustainable development and must be a top priority for the international community if some dent is to be made in reducing current inequities.
Formulate policy at all levels, from global to village scale, with greater awareness of the substantial inequalities in access to energy services that now exist, not only between countries but between populations within the same country and even between households within the same town or village.
In many developing countries, a small elite uses energy in much the same way as in the industrialized world, while most of the rest of the population relies on traditional, often poor-quality and highly polluting forms of energy. In other developing countries, energy consumption by a growing middle class is contributing significantly to global energy demand growth and is substantially raising national per capita consumption rates, despite little change in the consumption patterns of the very poor.
The reality that billions of people suffer from limited access to electricity and clean cooking fuels must not be lost in per capita statistics.
NEEDED ACTIONS
- Given the international dimension of the problem, multinational organizations like the United Nations and the World Bank should take the initiative to draw up a plan for eliminating the energy poverty of the world’s poor. As a first step, governments and NGOs can assist by supplying data on the extent of the problem in their countries.
- The private sector and the S&T community can help promote the transfer of appropriate technologies. The private sector can, in addition, help by making appropriate investments.
- The media should make the general public aware of the enormity of the problem.
Conclusion 2: improving energy efficiency and reducing carbon intensity
Concerted efforts must be made to improve energy efficiency and reduce the carbon intensity of the world economy. Economic competitiveness, energy security, and environmental considerations all argue for pursuing cost-effective, end-use efficiency opportunities. Such opportunities may be found throughout industry, transportation, and the built environment. To maximize efficiency gains and minimize costs, improvements should be incorporated in a holistic manner and from the ground up wherever possible, especially where long-lived infrastructure is involved. At the same time, it will be important to avoid underestimating the difficulty of achieving nominal energy efficiency gains, as frequently happens when analyses assume that reduced energy use is an end in itself rather than an objective regularly traded against other desired attributes.
RECOMMENDATIONS
Promote the enhanced dissemination of technology improvement and innovation between industrialized and developing countries. It will be especially important for all nations to work together to ensure that developing countries adopt cleaner and more efficient technologies as they industrialize.
Align economic incentives—especially for durable capital investments—with long-run sustainability objectives and cost considerations. Incentives for regulated energy service providers should be structured to encourage co-investment in cost-effective efficiency improvements, and profits should be delinked from energy sales.
Adopt policies aimed at accelerating the worldwide rate of decline in the carbon intensity of the global economy, where carbon intensity is measured as carbon dioxide equivalent emissions divided by gross world product, a crude measure of global well-being. Specifically, the Study Panel recommends immediate policy action to introduce meaningful price signals for avoided greenhouse gas emissions. Less important than the initial prices is that clear expectations be established concerning a predictable escalation of those prices over time. Merely holding carbon dioxide emissions constant over the next several decades implies that the carbon intensity of the world economy needs to decline at roughly the same rate as gross world product grows—achieving the absolute reductions in global emissions needed to stabilize atmospheric concentrations of greenhouse gases will require the worldwide rate of decline in carbon intensity to begin outpacing worldwide economic growth.
Enlist cities as a major driving force for the rapid implementation of practical steps to improve energy efficiency.
Inform consumers about the energy-use characteristics of products through labeling and implement mandatory minimum efficiency standards for appliances and equipment. Standards should be regularly updated and must be effectively enforced.
NEEDED ACTIONS
- Governments, in a dialogue with the private sector and the S&T community, should develop and implement (further) policies and regulations aimed at achieving greater energy efficiency and lower energy intensity for a great variety of processes, services, and products.
- The general public must be made aware, by governments, the media, and NGOs of the meaning and necessity of such policies and regulations.
- The S&T community should step up its efforts to research and develop new, low-energy technologies.
- Governments, united in intergovernmental organizations, should agree on realistic price signals for carbon emissions—recognizing that the economies and energy systems of different countries will result in different individual strategies and trajectories—and make these price signals key components of further actions on reducing the carbon emissions.
- The private sector and the general public should insist that governments issue clear carbon price signals.
Conclusion 3: carbon capture and storage technologies needed, including carbon-negative bioenergy
Technologies for capturing and sequestering carbon from fossil fuels, particularly coal, can play a major role in the cost-effective management of global carbon dioxide emissions. As the world’s most abundant fossil fuel, coal will continue to play a large role in the world’s energy mix. It is also the most carbon-intensive conventional fuel in use, generating almost twice as much carbon dioxide per unit of energy supplied than natural gas. Today, new coal-fired power plants—most of which can be expected to last more than half a century—are being constructed at an unprecedented rate. Moreover, the carbon contribution from coal could expand further if nations with large coal reserves like the United States, China, and India turn to coal to address energy security concerns and develop alternatives to petroleum.
RECOMMENDATIONS
Accelerate the development and deployment of advanced coal technologies. Without policy interventions the vast majority of the coal-fired power plants constructed in the next two decades will be conventional, pulverized coal plants. Present technologies for capturing carbon dioxide emissions from pulverized coal plants on a retrofit basis are expensive and energy intensive. Where new coal plants without capture must be constructed, the most efficient technologies should be used. In addition, priority should be given to minimize the costs of future retrofits for carbon capture by developing at least some elements of carbon capture technology at every new plant. Active efforts to develop such technologies for different types of base plants are currently underway and should be encouraged by promoting the construction of full-scale plants that utilize the latest technology advances.
Aggressively pursue efforts to commercialize carbon capture and storage. Moving forward with full-scale demonstration projects is critical, as is continued study and experimentation to reduce costs, improve reliability, and address concerns about leakage, public safety, and other issues. For capture and sequestration to be widely implemented, it will be necessary to develop regulations and to introduce price signals for carbon emissions. Based on current cost estimates, the Study Panel believes price signals on the order of US$100–150 per avoided metric ton of carbon equivalent (US$27–41 per ton of carbon dioxide equivalent) will be required to induce the widespread adoption of carbon capture and storage. Price signals at this level would also give impetus to the accelerated deployment of biomass and other renewable energy technologies.
Explore potential retrofit technologies for post-combustion carbon capture suitable for the large and rapidly growing population of existing pulverized coal plants. In the near term, efficiency improvements and advanced pollution control technologies should be applied to existing coal plants as a means of mitigating their immediate climate change and public health impacts.
Pursue carbon capture and storage with systems that co-fire coal and biomass. This technology combination provides an opportunity to achieve net negative greenhouse gas emissions—effectively removing carbon dioxide from the atmosphere (Note: this is a combination of technologies the Biopact has been striving to disemminate - earlier post).
NEEDED ACTIONS
- The private sector and the S&T community should join forces to further investigate the possibilities for carbon capture and sequestration and develop adequate technologies for demonstration.
- Governments should facilitate the development of these technologies by making available funds and opportunities (such as test sites).
- The general public needs to be thoroughly informed about the advantages of carbon sequestration and about the relative manageability of associated risks. The media can assist with this.
Conclusion 4: alternatives to oil and gas
Competition for oil and natural gas supplies has the potential to become a source of growing geopolitical tension and economic vulnerability for many nations in the decades ahead. In many developing countries, expenditures for energy imports also divert scarce resources from other urgent public health, education, and infrastructure development needs. The transport sector accounts for just 25 percent of primary energy consumption worldwide, but the lack of fuel diversity in this sector makes transport fuels especially valuable.
RECOMMENDATIONS
Introduce policies and regulations that promote reduced energy consumption in the transport sector by (a) improving the energy efficiency of automobiles and other modes of transport and (b) improving the efficiency of transport systems (e.g., through investments in mass transit, better land-use and city planning, etc.).
Develop alternatives to petroleum to meet the energy needs of the transport sector, including biomass fuels, plug-in hybrids, and compressed natural gas, as well as — in the longer run — advanced alternatives, such as hydrogen fuel cells.
Implement policies to ensure that the development of petroleum alternatives is pursued in a manner that is compatible with other sustainability objectives. Current methods for liquefying coal and extracting oil from unconventional sources, such as tar sands and shale oil, generate substantially higher levels of carbon dioxide and other pollutant emissions compared to conventional petroleum consumption. Even with carbon capture and sequestration, a liquid fuel derived from coal will at best produce emissions of carbon dioxide roughly equivalent to those of conventional petroleum at the point of combustion. If carbon emissions from the conversion process are not captured and stored, total fuel-cycle emissions for this energy pathway as much as double. The conversion of natural gas to liquids is less carbon intensive than coal to liquids, but biomass remains the only near-term feedstock that has the potential to be truly carbon-neutral and sustainable on a long-term basis. In all cases, full fuel-cycle impacts depend critically on the feedstock being used and on the specific extraction or conversion methods being employed.
NEEDED ACTIONS
- Governments should introduce (further) policies and regulations aimed at reducing energy consumption and developing petroleum alternatives for use in the transport sector.
- The private sector and the S&T community should continue developing technologies adequate to that end.
- The general public’s awareness of sustainability issues related to transportation energy use should be significantly increased. The media can play an important role in this effort.
Conclusion 5: nuclear power welcomed, but with caution
As a low-carbon resource, nuclear power can continue to make a significant contribution to the world’s energy portfolio in the future, but only if major concerns related to capital cost, safety, and weapons proliferation are addressed. Nuclear power plants generate no carbon dioxide or conventional air pollutant emissions during operation, use a relatively abundant fuel feedstock, and involve orders-of-magnitude smaller mass flows, relative to fossil fuels. Nuclear’s potential, however, is currently limited by concerns related to cost, waste management, proliferation risks, and plant safety (including concerns about vulnerability to acts of terrorism and concerns about the impact of neutron damage on plant materials in the case of life extensions). A sustained role for nuclear power will require addressing these hurdles.
RECOMMENDATIONS
Replace the current fleet of aging reactors with plants that incorporate improved intrinsic (passive) safety features.
Address cost issues by pursuing the development of standardized reactor designs.
Understand the impact of long-term aging on nuclear reactor systems (e.g., neutron damage to materials) and provide for the safe and economic decommissioning of existing plants.
Develop safe, retrievable waste management solutions based on dry cask storage as longer-term disposal options are explored. While long-term disposal in stable geological repositories is technically feasible, finding socially acceptable pathways to implement this solution remains a significant challenge.
Address the risk that civilian nuclear materials and knowledge will be diverted to weapons applications (a) through continued research on proliferation-resistant uranium enrichment and fuel-recycling capability and on safe, fast neutron reactors that can burn down waste generated from thermal neutron reactors and (b) through efforts to remedy shortcomings in existing international frameworks and governance mechanisms.
Undertake a transparent and objective re-examination of the issues surrounding nuclear power and their potential solutions. The results of such a reexamination should be used to educate the public and policymakers.
NEEDED ACTIONS
- Given the controversy over the future of nuclear power worldwide, the United Nations should commission—as soon as possible—a transparent and objective re-examination of the issues that surround nuclear power and their potential solutions. It is essential that the general public be informed about the outcome of this re-examination.
- The private sector and the S&T community should continue research and development efforts targeted at improving reactor safety and developing safe waste management solutions.
- Governments should facilitate the replacement of the current fleet of aging reactors with modern, safer plants. Governments and intergovernmental organizations should enhance their efforts to remedy shortcomings in existing international frameworks and governance mechanisms.
Conclusion 6: supporting non-biomass renewables
Renewable energy in its many forms offers immense opportunities for technological progress and innovation. Over the next 30–60 years, sustained efforts must be directed toward realizing these opportunities as part of a comprehensive strategy that supports a diversity of resource options over the next century. The fundamental challenge for most renewable options involves cost-effectively tapping inherently diffuse and in some cases intermittent resources. Sustained, long-term support—in various forms—is needed to overcome these hurdles. Renewable energy development can provide important benefits in underdeveloped and developing countries because oil, gas, and other fuels are hard cash commodities.
RECOMMENDATIONS
Implement policies—including policies that generate price signals for avoided carbon emissions—to ensure that the environmental benefits of renewable resources relative to non-renewable resources will be systematically recognized in the marketplace.
Provide subsidies and other forms of public support for the early deployment of new renewable technologies. Subsidies should be targeted to promising but not-yet-commercial technologies and decline gradually over time.
Explore alternate policy mechanisms to nurture renewable energy technologies, such as renewable portfolio standards (which set specific goals for renewable energy deployment) and ‘reverse auctions’ (in which renewable energy developers bid for a share of limited public funds on the basis of the minimum subsidy they require on a per kilowatt-hour basis).
Invest in research and development on more transformational technologies, such as new classes of solar cells that can be made with thin-film, continuous fabrication processes.
Conduct sustained research to assess and mitigate any negative environmental impacts associated with the large-scale deployment of renewable energy technologies. Although these technologies offer many environmental benefits, they may also pose new environmental risks as a result of their low power density and the consequently large land area required for large-scale deployment.
NEEDED ACTIONS
- Governments should substantially facilitate the use—in an environmentally sustainable way—of renewable energy resources through adequate policies and subsidies. A major policy step in this direction would include implementing clear price signals for avoided greenhouse gas emissions.
- Governments should also promote research and development in renewable energy technologies by supplying significantly more public funding.
- The private sector, aided by government subsidies, should seek entrepreneurial opportunities in the growing renewable energy market.
- The S&T community should devote more attention to overcoming the cost and technology barriers that currently limit the contribution of renewable energy sources.
- NGOs can assist in promoting the use of renewable energy sources in developing countries.
- The media can play an essential role in heightening the general public’s awareness of issues related to renewable energy.
Conclusion 7: biofuels hold great promise for simultaneously addressing climate-change and energy-security concerns
Improvements in agriculture will allow for food production adequate to support a predicted peak world population on the order of 9 billion people with excess capacity for growing energy crops. Maximizing the potential contribution of biofuels requires commercializing methods for producing fuels from lignocellulosic feedstocks (including agricultural residues and wastes), which have the potential to generate five to ten times more fuel than processes that use starches from feedstocks, such as sugar cane and corn. Recent advances in molecular and systems biology show great promise in developing improved feedstocks and much less energy-intensive means of converting plant material into liquid fuel. In addition, intrinsically more efficient conversion of sunlight, water, and nutrients into chemical energy may be possible with microbes.
RECOMMENDATIONS
Conduct intensive research into the production of biofuels based on lignocellulose conversion.
Invest in research and development on direct microbial production of butanol or other forms of biofuels that may be superior to ethanol.
Implement strict regulations to insure that the cultivation of biofuels feedstocks accords with sustainable agricultural practices and promotes biodiversity, habitat protection, and other land management objectives.
Develop advanced bio-refineries that use biomass feedstocks to self-generate power and extract higher-value co-products. Such refineries have the potential to maximize economic and environmental gains from the use of biomass resources.
Develop improved biofuels feedstocks through genetic selection and/or molecular engineering, including drought resistant and self-fertilizing plants that require minimal tillage and fertilizer or chemical inputs.
Mount a concerted effort to collect and analyze data on current uses of biomass by type and technology (both direct and for conversion to other fuels), including traditional uses of biomass.
Conduct sustained research to assess and mitigate any adverse environmental or ecosystem impacts associated with the large-scale cultivation of biomass energy feedstocks, including impacts related to competition with other land uses (including uses for habitat preservation and food production), water needs, etc.
NEEDED ACTIONS
- The S&T community and the private sector should greatly augment their research and development (and deployment) efforts toward more efficient, environmentally sustainable technologies and processes for the production of modern biofuels.
- Governments can help by stepping up public research and development funding and by adapting existing subsidy and fiscal policies so as to favor the use of biofuels over that of fossil fuels, especially in the transport sector.
- Governments should pay appropriate attention to promoting sustainable means of biofuels production and to avoiding conflicts between biofuel production and food production.
Conclusion 8: development of new infrastructures
The development of cost-effective energy storage technologies, new energy carriers, and improved transmission infrastructure could substantially reduce costs and expand the contribution from a variety of energy supply options.
Such technology improvements and infrastructure investments are particularly important to tap the full potential of intermittent renewable resources, especially in cases where some of the most abundant and cost-effective resource opportunities exist far from load centers. Improved storage technologies, new energy carriers, and enhanced transmission and distribution infrastructure will also facilitate the delivery of modern energy services to the world’s poor—especially in rural areas.
RECOMMENDATIONS
Continue long-term research and development into potential new energy carriers for the future, such as hydrogen. Hydrogen can be directly combusted or used to power a fuel cell and has a variety of potential applications, including as an energy source for generating electricity or in other stationary applications and as an alternative to petroleum fuels for aviation and road transport. Cost and infrastructure constraints, however, are likely to delay widespread commercial viability until mid-century or later.
Develop improved energy storage technologies, either physical (e.g., compressed air or elevated water storage) or chemical (e.g., batteries, hydrogen, or hydrocarbon fuel produced from the reduction of carbon dioxide) that could significantly improve the market prospects of intermittent renewable resources, such as wind and solar power.
Pursue continued improvements and cost reductions in technologies for transmitting electricity over long distances. High-voltage, direct-current transmission lines, in particular, could be decisive in making remote areas accessible for renewable energy development, improving grid reliability, and maximizing the contribution from a variety of low-carbon electricity sources. In addition, it will be important to improve overall grid management and performance through the development and application of advanced or ‘smart’ grid technologies that could greatly enhance the responsiveness and reliability of electricity transmission and distribution networks.
NEEDED ACTIONS
- The S&T community, together with the private sector, should have focus on research and development in this area.
- Governments can assist by increasing public funding for research and development and by facilitating needed infrastructure investments.
Conclusion 9: S&T community needs to be engaged
The S&T community — together with the general public — has a critical role to play in advancing sustainable energy solutions and must be effectively engaged.
As noted repeatedly in the foregoing recommendations, the energy challenges of this century and beyond demand sustained progress in developing, demonstrating, and deploying new and improved energy technologies. These advances will need to come from the S&T community, motivated and supported by appropriate policies, incentives, and market drivers.
RECOMMENDATIONS
Provide increased funding for public investments in sustainable energy research and development, along with incentives and market signals to promote increased private-sector investments.
Effect greater coordination of technology efforts internationally, along with efforts to focus universities and research institutions on the sustainability challenge.
Conduct rigorous analysis and scenario development to identify possible combinations of energy resources and end-use and supply technologies that have the potential to simultaneously address the multiple sustainability challenges linked to energy.
Stimulate efforts to identify and assess specific changes in institutions, regulations, market incentives, and policy that would most effectively advance sustainable energy solutions.
Create an increased focus on specifically energy-relevant awareness, education, and training across all professional fields with a role to play in the sustainable energy transition.
Initiate concerted efforts to inform and educate the public about important aspects of the sustainable energy challenge, such as the connection between current patterns of energy production and use and critical environmental and security risks.
Begin enhanced data collection efforts to support better decision-making in important policy areas that are currently characterized by a lack of reliable information (large cities in many developing countries, for example, lack the basic data needed to plan effectively for transportation needs).
The S&T community must strive for better international coordination of energy research and development efforts, partly in collaboration with the private sector. It should seek to articulate a focused, collaborative agenda aimed at addressing key obstacles to a sustainable energy future.
NEEDED ACTIONS
- Governments (and intergovernmental organizations) must make more public funding available to not only boost the existing contribution from the S&T community but also to attract more scientists and engineers to working on sustainable energy problems.
- The why and how of energy research and development should be made transparent to the general public to build support for the significant and sustained investments that will be needed to address long-term sustainability needs.
- The S&T community itself, intergovernmental organizations, governments, NGOs, the media, and—to a lesser extent—the private sector should be actively engaged in educating the public about the need for these investments.
Lighting the Way: it can be done
While the current energy outlook is very sobering, the Study Panel believes that there are sustainable solutions to the energy problem. Aggressive support of energy science and technology must be coupled with incentives that accelerate the concurrent development and deployment of innovative solutions that can transform the entire landscape of energy demand and supply. Opportunities to substitute superior supply-side and end-use technologies exist throughout the world’s energy systems, but current investment flows generally do not reflect these opportunities.
Science and engineering provide guiding principles for the sustainability agenda. Science provides the basis for a rational discourse about trade-offs and risks, for selecting research and development priorities, and for identifying new opportunities—openness is one of its dominant values. Engineering, through the relentless optimization of the most promising technologies, can deliver solutions—learning by doing is among its dominant values. Better results will be achieved if many avenues are explored in parallel, if outcomes are evaluated with actual performance measures, if results are reported widely and fully, and if strategies are open to revision and adaptation.
Long-term energy research and development is thus an essential component of the pursuit of sustainability. Significant progress can be achieved with existing technology but the scale of the long-term challenge will demand new solutions. The research community must have the means to pursue promising technology pathways that are already in view and some that may still be over the horizon.
The transition to sustainable energy systems also requires that market incentives be aligned with sustainability objectives. In particular, robust price signals for avoided carbon emissions are critical to spur the development and deployment of low-carbon energy technologies. Such price signals can be phased in gradually, but expectations about how they will change over time must be established in advance and communicated clearly so that businesses can plan with confidence and optimize their long-term capital investments.
Critical to the success of all the tasks ahead are the abilities of individuals and institutions to effect changes in energy resources and usage. Capacity building, both in terms of investments in individual expertise and institutional effectiveness, must become an urgent priority of all principal actors: multi-national organizations, governments, corporations, educational institutions, non-profit organizations, and the media. Above all, the general public must be provided with sound information about the choices ahead and the actions required for achieving a sustainable energy future.
Background
In February 2005, at the request of the Governments of China and Brazil, and with strong support from then-United Nations Secretary-General Mr. Kofi Annan, the InterAcademy Council Board decided to launch an in-depth study on how to achieve global transitions to an adequately affordable, sustainable, clean energy supply.
After appointing the panel—which includes experts from Brazil, China, Europe, India, Japan, Russia, the US and other nations—the IAC commissioned 19 reports to inform seven energy workshops held in 2005 and 2006. The report underwent an extensive, monitored peer review and incorporates the analysis and actions of leading global energy and development institutions, such as the United Nations Development Program, the World Bank and the International Energy Agency.
There are three key questions addressed in the study:
- How can we provide universal access to affordable, modern power?
- What is the most efficient way to address environmental costs?
- How can we establish energy security?
The Organizing Group advised the IAC to commission a total of 19 papers on various topics considered important for the study, as “intellectual start capital” for the Study Panel. This advice was carried out; 16 papers were received and used as background/discussion material in workshops.
Taking into consideration nominations from science and engineering academies and advice from the Organizing Group, the IAC Board formally approved in September 2005 a slate of candidates. Fifteen persons were subsequently appointed to the Study Panel:
Two Study Panel Co-Chairs:
- Steven Chu (USA), Director, Lawrence Berkeley National Laboratory & Professor of Physics and Professor of Molecular and Cell Biology, University of California, Berkeley
- José Goldemberg (Brazil), Professor of the University of São Paulo, Brazil
Thirteen Study Panel Members were: Shem Arungu Olende (Kenya), Secretary-General, African Academy of Sciences & Chairman and Chief Executive Officer, Queconsult Ltd; Ged Davis (UK), Co-President, Global Energy Assessment, International Institute for Applied Systems Analysis (IIASA); Mohamed El-Ashry (Egypt), Senior Fellow, UN Foundation; Thomas B. Johansson (Sweden), Professor of Energy Systems Analysis and Director of the International Institute for Industrial Environmental Economics (IIIEE) at Lund University; David Keith (Canada), Director, ISEEE Energy and Environmental Systems Group & Professor and Canada Research Chair in Energy and the Environment, University of Calgary; Li Jinghai (China), Vice President of the Chinese Academy of Sciences; Nebosja Nakicenovic (Austria), Professor of Energy Economics at Vienna University of Technology & Leader of Energy and Technology Programs at IIASA (International Institute for Applied Systems Analysis); Rajendra Pachauri (India), Director-General, The Energy and Resources Institute & Chairman, Intergovernmental Panel on Climate Change (2007 Nobel Peace Prize Winner); Majid Shafie-Pour (Iran), Professor of Environmental Engineering (Energy, Air Pollution and Climate Change), Faculty of Environment, University of Tehran; Evald Shpilrain (Russia), Professor of Thermophysics and Renewable Energy Sources, Corresponding member of the Russian Academy of Sciences (RAS), Advisor to the RAS; Robert Socolow (USA), Professor of Mechanical and Aerospace Engineering at Princeton University; Kenji Yamaji (Japan), Professor of Electrical Engineering at the University of Tokyo, Member of Science Council of Japan, Vice-Chair of IIASA Council & Chairman of the Green Power Certification Council of Japa; and Yan Luguang (China), Chairman of the Scientific Committee of Institute of Electrical Engineering, Chinese Academy of Sciences & Honorary President of Ningbo University.
The work of the Study Panel was assisted by Jos van Renswoude, Study Director; Dilip Ahuja, Professor, Indian National Institute of Advanced Studies, as Special Advisor to the Study Panel; and Marika Tatsutani, writer and editor.
The Study Panel convened seven workshops—most hosted by national scientific academies— to obtain additional insights into energy issues facing different regions of the world.
Financial contributions for this study have been gratefully received from the Chinese Academy of Sciences, the Government of Brazil, the William and Flora Hewlett Foundation, the United Nations Foundation, the Deutsche Forschungsgemeinschaft, and the Energy Foundation.
References:
InterAcademy Council: Lighting the Way: Toward a Sustainable Energy Future - October 2007.
The Study Panel convened seven workshops - most hosted by national scientific academies — to obtain additional insights into energy issues facing different regions of the world. For more information on the workshops, click here.
An international teleconference [*.mp3] was held Monday, October 22 at 2 PM GMT for reporters to address questions to members of the IAC study panel that wrote the new report 'Lighting the Way: Toward a Sustainable Energy Future.'
Article continues
Tuesday, October 23, 2007
Growth in carbon emissions accelerating; exceeding worst case scenario
The growth of carbon emissions from fossil fuels has tripled compared to the 1990s and is now exceeding the predictions of the highest IPCC emission scenarios - the problematic A1FI scenario, based on very rapid, fossil intensive economic growth (graph, click to enlarge). Key data from the report:
- Atmospheric CO2 has grown at 1.9 parts per million (ppm) per year compared to about 1.5 ppm during the previous 30 years
The growth in emissions from fossil fuels increased from 1.3% per year for 1990-1999 to 3.3% per year during the period running from 2000 to 2006; total carbon emissions now stand at 9.9 Petagram per year, of which 8.4 comes from fossil fuels and 1.5 Pg from land-use changes (graph, click to enlarge)- Emissions generated by land-use changes have remained constant, but have shifted in geographic focus (from South America, where a decline in deforestation has occured, to South and South East Asia)
The efficiency of natural carbon sinks - oceans and terrestrial plant growth - has decreased by 10% over the last 50 years and will continue to do so in the future, implying that the longer we wait to reduce emissions, the larger the cuts needed to stabilize atmospheric CO2. A number of large droughts in the mid-latitudes (Europe, North America, Central asia) has reduced the capacity of plants to sequester carbon in these regions (map, click to enlarge)
For the first time in 100 years, the carbon intensity of the world’s economy has stopped decreasing; since 2000, carbon output per dollar of productivity has been increasing by 0.3% per year, whereas during the previous 3 decades, it was dropping by an average of 1.3% per year (graph, click to enlarge)- All of these changes characterize a carbon cycle that is generating stronger climate forcing and sooner than expected
The share of the different drivers of the increased atmospheric CO2 levels is as follows:Between 2000 to 2006, human activities such as burning fossil fuels, manufacturing cement, and land-use changes contributed an average of 4.1 billion metric tons of carbon to the atmosphere each year, yielding an annual growth rate for atmospheric carbon dioxide of 1.93 ppm. This is the highest since the beginning of continuous monitoring in 1959, states the report. The growth rate of atmospheric carbon dioxide is significantly larger than those for the 1980s and 1990s, which were 1.58 and 1.49 ppm per year, respectively. The present atmospheric concentration of carbon dioxide is 381 ppm, the largest concentration in the last 650,000 years, and probably in the last 20 million years:
While the worldwide acceleration in carbon dioxide emissions had been previously noted, the current analysis provides insights into its causes. “The new twist here is the demonstration that weakening land and ocean sinks are contributing to the accelerating growth of atmospheric CO2,” says co-author Chris Field, director of the Carnegie Institution’s Department of Global Ecology.
Changes in wind patterns over the Southern Ocean resulting from human-induced global warming have brought carbon-rich water toward the surface, reducing the ocean’s ability to absorb excess carbon dioxide from the atmosphere. On land, where plant growth is the major mechanism for drawing carbon dioxide out of the atmosphere, large droughts have reduced the uptake of carbon.
Emissions from the burning of fossil fuels constituted the largest source of anthropogenic carbon, releasing an average of 7.6 billion metric tons each year between 2000 and 2006, a significant jump from 6.5 billion tons in the 1990s. Emissions generated by land-use changes such as deforestation have remained constant, but shifted in geographic focus.
The study also shows that the carbon intensity of the global economy (kilograms of carbon per dollar of economic activity) has increased since 2000 at about 0.3% per year, reversing a 30-year decline of about 1.3% per year. Because practically all proposed scenarios for managing future emissions postulate improvements in carbon intensity in the global economy, this deterioration of carbon intensity presents a serious challenge in stabilizing atmospheric carbon dioxide and mitigating climate change.
The paper presents "a consistent picture of the increasing accumulation of atmospheric CO2 and, hence, the increasing urgency to do something about it," says physical scientist S. Randy Kawa of NASA's Goddard Space Flight Center in Greenbelt, Maryland. But he cautions about jumping to long-term conclusions. "Just because the last 7 years have shown accelerating trends does not mean that the next 7 or 50 or 100 will be the same," Kawa says. "But they are what they are, and we need to pay attention."
The research team included scientists from the following institutions: CSIRO Marine and Atmospheric Research (Australia); the International Institute for Applied Systems Analysis, Laxenburg (Austria); the Commissariat a L'Energie Atomique, Laboratorie des Sciences du Climat et de l'Environnement (France); University of East Anglia, School of Environment Sciences, Norwich, (UK), the British Antarctic Survey, Cambridge (UK); the Carnegie Institution of Washington, Department of Global Ecology, Stanford (USA); the NOAA Earth System Research Laboratory, Boulder (USA); the Woods Hole Research Center, Falmouth (USA); and the Carbon Dioxide Information Analysis Center, Oak Ridge National Laboratory, Oak Ridge (USA).
References:
Michael R. Raupach, Gregg Marland, Philippe Ciais, Corinne Le Quéré, Josep G. Canadell, Gernot Klepper, and Christopher B. Field, "Global and regional drivers of accelerating CO2 emissions", PNAS, June 12, 2007, vol. 104, no. 24, 10288-10293
Eurekalert: Rise in atmospheric CO2 accelerates as economy grows, natural carbon sinks weaken - October 22, 2007.
GLobal Carbon Project: "Recent Carbon Trends and the Global Carbon Budget updated to 2006" [*.pdf], GCP-Global Carbon Budget team: Pep Canadell, Philippe Ciais, Thomas Conway, Chris Field, Corinne Le Quéré, Skee Houghton, Gregg Marland, Mike Raupach, Erik Buitenhuis, Nathan Gillett; Last update: 20 October 2007
The 6 emissions scenarios developed by the International Panel on Climate Change and still used as the reference can be found here: IPPC Special Report: Emissons Scenarios, Summary for Policy Makers [*.pdf] - 2000.
Article continues
posted by Biopact team at 5:47 PM 0 comments links to this post