Back to black: hydrothermal carbonisation of biomass to clean up CO2 emissions from the past

Cleaning up the past: going carbon-negative
A growing number of scientists however is looking at ways to clean up our emissions from the past. In order to avert 'catastrophic' climate change, it has become desirable to invert the current development by sequestering the atmospheric CO2 of the past 200 years of industrialization. Some have suggested the rapid implementation of geo-engineering options, such as building vast forests of artificial trees that can suck CO2 out of the atmosphere and store it in geological formations (such as depleted coal or gas fields or saline aquifers). Such 'synthetic trees' would however be quite costly and will not deliver energy as they do their work (earlier post).
Gradually, a new, 'low-tech' geosequestration option is attracting the attention of more and more scientists. It is based on converting biomass into an inert form of bio-coal or charcoal, that can be stored in soils. Earlier we referred to carbon-negative energy systems that rely on gasification and biochar sequestration: biomass is gasified which results in a carbon monoxide and hydrogen rich gas that can be used for energy or transformed into ultra-clean synthetic biofuels via the Fischer-Tropsch process, whereas a fraction becomes bio-char that can be stored in soils (using a technique known as 'terra preta'). Similar techniques can be build around pyrolysis processes (earlier post). In such systems, soil fertility would be gradually enhanced, 'historic' CO2 would be sequestered and clean biofuels could be used to power our societies.
Only biomass can be used for the creation of such carbon-negative energy systems that clean up our emissions from the past. Other renewables are carbon-neutral at best, meaning they can only reduce future CO2 emissions - something many scientists think is not enough to avert dangerous climate change.
Maria-Magdalena Titirici, Arne Thomas and Markus Antonietti of the Department of Colloid Chemistry at the Max Planck Institute of Colloids and Interfaces, now describe a new, highly efficient though 'low-tech' way to use biomass as a tool to clean up past emissions. Their research appears in an open access article in the New Journal of Chemistry, in which they suggest creating "turbo-rainforests" based on fast-growing energy crops that are grown, turned into bio-coal via a process known as hydrothermal carbonization (HTC), and then stored into 'carbon landfills', while deriving energy from the process. The technique can be practised on an ultra-large scale, and can thus be described as a geo-engineering option - one that is actually technically and economically feasible.
Importantly, in contrast to other biomass carbonisation techniques that require dry biomass, the hydrothermal carbonisation process is a highly efficient 'wet' process that avoids complicated drying schemes and costly isolation procedures. The resulting carbonaceous materials also open a new field of chemistry, full of novel possibilities and challenges that may lead to the development of new (nano)materials:

Biomass as a carbon converter
The biggest carbon converter, with the highest efficiency to bind CO2 from the atmosphere, is certainly biomass, the scientists write. A rough estimate of terrestrial biomass growth amounts to 118 × 109 tons per year, when calculated as dry matter. Biomass, however, is just a short term, temporary carbon sink, as microbial decomposition liberates exactly the amount of CO2 formerly bound in the plant material:
Nevertheless, as biomass contains about 0.4 mass equivalents of carbon, removal of 8.5% of the freshly produced biomass from the active geosystem would indeed compensate for the complete CO2 liberation from oil, all numbers calculated per year.To make biomass effective as a carbon sink, the carbon in it has to be fixed by low-tech operations. Coal formation is certainly one of the natural sinks that has been active in the past on the largest scale. Natural coalification of biomass takes place on a timescale of hundred million years. Due to its slowness, it is usually not considered in renewable energy exploitation schemes or as an active sink in CO2 cycles. Nevertheless, it is obvious that carbon fixation into coal is a lasting effort, as brown or black coal (on the contrary to peat) are obviously practically not biodegradable. The question of coalified carbon destabilization is, however, currently accessed in more detail. Sufficient condensation of the carbon scaffold is, in any case, mandatory for the purposes of carbon fixation.
It is therefore the purpose of this contribution to discuss the feasibility of turning coal formation into an active element of carbon sequestration schemes, simply by accelerating the underlying coalification processes by chemical means. The natural process of peat or coal formation is presumably not biological but chemical in its nature. As coaling is a rather elemental experiment, coals and tars have been made and used by mankind since the Stone Age, and one can find trials to imitate carbon formation from carbohydrates with faster chemical processes in the modern scientific literature. In this context, it is an exciting observation of soil research that the Indians of the Amazon basin used locally generated charcoal for the improvement of soil quality for hundreds of years (i.e. improving the water and ion binding of rich black soil) and that this carbon fraction was not easily decomposed.
Hydrothermal carbonisation
Besides charcoal formation, which is performed with high quality, dry biomass only, hydrothermal carbonization (HTC) is an especially promising process. The first experiments were carried out by Bergius, who, in 1913, had already described the hydrothermal transformation of cellulose into coal-like materials.9 More systematic investigations were performed by Berl and Schmidt in 1932, which varied the biomass source and treated the different samples, in the presence of water, at temperatures between 150 and 350 °C.10 The latter authors summarized, via a series of papers in 1932, the knowledge of those days about the emergence of coal. Later, Schuhmacher et al. analyzed the influence of pH on the outcome of the HTC reaction and found serious differences in the decomposition schemes, as identified by the C/H/O composition.
A renaissance in such experiments was started with reports on the low temperature hydrothermal synthesis of carbon spheres (highter than or equal to 200°C), and gave exciting nanostructures. It was also revealed that the presence of ternary components in complex biomass (such as orange peel or oak leaves) seriously alters decomposition schemes. Unexpectedly, an improvement in properties of the carbonaceous structures for certain applications was found, i.e. smaller structural size of carbon dispersions and porous networks, higher hydrophilicity of the surfaces, and higher capillarity.
For completeness, it must also be mentioned that, beyond coalification, the conversion of biomass under hydrothermal conditions is a widely examined process. These approaches aim for the recovery of liquid or gaseous fuel intermediates (like glucose, 5-hydroxymethylfurfural, methane, hydrogen etc.) from biomass, while the solid residues were, up until now, mostly treated as undesirable side products.
However, the described acceleration of HTC for coalification by a factor of 106–109 under rather soft conditions, down to a scale of hours, also makes it a considerable, technically-attractive alternative for the sequestration of carbon from biomass on large and ultra-large scales. Finally, to summarize the outcome of the optimization trials, catalyzed HTC required only the heating of a biomass dispersion under weakly acidic conditions in a closed reaction vessel for 4–24 h to temperatures of around 200 °C. This is indeed an extremely simple, cheap and easily scalable process. Besides that, HTC has a number of other practical advantages. HTC inherently requires wet starting products or biomass, as effective dehydration only occurs in the presence of water, plus the final carbon can be easily filtered from the reaction solution. This way, complicated drying schemes and costly isolation procedures can be conceptually avoided. In addition, under acidic conditions and below 200 °C, most of the original carbon stays bound to the final structure. Carbon structures produced by this route—either for deposit or materials use—are therefore the most CO2-efficient.

Carbon storage
Therefore, we strongly believe that the carbonization of fast growing plants is currently the most efficient process for removing atmospheric CO2, binding it into depositable carbon or even as useful solids.
For a negative atmospheric CO2 balance, the generated carbonaceous materials have to be deposited on a large scale, and potential carbon landfills may lay the foundations for chemical starting materials of the next century.
Another quite attractive application with immediate impact is their use as water- and ion-binding components to improve soil quality. This is a chemical process that is also found in nature, and carbonaceous soil is presumably the largest active carbon sink on earth. The proposed terra preta, i.e. artificial coal-enriched soil as a potential carbon sink of global dimensions, has already been mentioned in soil research, improving soil quality and plant growth at the same time. Instead of clearing the rainforest for questionable palm oil production, such a carbon-reinforced "turbo-rainforest" would produce at least 10 times the energy, but stored in carbon, whilst also being CO2-negative for the climate and supporting biodiversity at the same time.
Spending just 10% of our oil expenses on global CO2 sequestration would compensate for carbon fixation costs of US$44 per ton, a target which can, in the researchers' opinion, be quite easily met (HTC is essentially just heating an aqueous dispersion, where even the energy is generated by the process itself) and does not even consider the added value for the geosystem or agriculture.
Even in industrial countries such as Germany, only the treatment of highly defined waste biomass, such as from sugar-beet (4.3 Mt sugar per year), rapeseed production (3.5 Mt oil per year), or clarification sludge (3.0 Mt per year), has the potential to lower German CO2 output by about 10%. The low-tech processing of fast growing plants into non- or weakly-degradable peat-type carbon scaffolds by hydrothermal reaction cascades is therefore, in our opinion, a realistic artificial instrument for reducing atmospheric CO2.
New chemistry
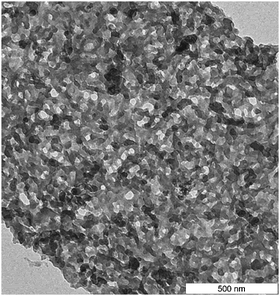
More information:
Maria-Magdalena Titirici, Arne Thomas and Markus Antonietti, "Back in the black: hydrothermal carbonization of plant material as an efficient chemical process to treat the CO2 problem?", New J. Chem., 8th March 2007, DOI: 10.1039/b616045j
2 Comments:
Terra Preta has gotten much good news since the IAI conference and recent major articles;
In Focus
May 15, 2007
Special Report: Inspired by Ancient Amazonians, a Plan to Convert Trash into Environmental Treasure
New bill in U.S. Senate will advocate adoption of "agrichar" method that could lessen our dependence on fossil fuel and help avert global warming
By Anne Casselman
http://www.sciam.com/article.cfm?articleID=5670236C-E7F2-99DF-3E2163B9FB144E40
The main hurtle now is to change the current perspective held by the IPCC that the soil carbon cycle is a wash, to one in which soil can be used as a massive and ubiquitous Carbon sink via Charcoal. Below are the first concrete steps in that direction;
Tackling Climate Change in the U.S.
Potential Carbon Emissions Reductions from Biomass by 2030
by Ralph P. Overend, Ph.D. and Anelia Milbrandt
National Renewable Energy Laboratory
http://www.ases.org/climatechange/toc/07_biomass.pdf
The organization 25x25 (see 25x'25 - Home) released it's (first-ever, 55-page )"Action Plan" ; see http://www.25x25.org/storage/25x25/d...ActionPlan.pdf
On page 31, as one of four foci for recommended RD&D, the plan lists: "The development of biochar, animal agriculture residues and other non-fossil fuel based fertilizers, toward the end of integrating energy production with enhanced soil quality and carbon sequestration."
and on p 32, recommended as part of an expanded database aspect of infrastructure: "Information on the application of carbon as fertilizer and existing carbon credit trading systems."
I feel 25x25 is now the premier US advocacy organization for all forms of renewable energy, but way out in front on biomass topics.
There are 24 billion tons of carbon controlled by man in his agriculture , I forgot the % that is waste, but when you add all the other cellulose waste which is now dumped to rot or digested or combusted and ultimately returned to the atmosphere as GHG, the balanced number is around 24 Billion tons. So we have plenty of bio-mass.
Even with all the big corporations coming to the GHG negotiation table, like Exxon, Alcoa, .etc, we still need to keep watch as they try to influence how carbon management is legislated in the USA. Carbon must have a fair price, that fair price and the changes in the view of how the soil carbon cycle now can be used as a massive sink verses it now being viewed as a wash, will be of particular value to farmers and a global cool breath of fresh air for us all.
If you have any other questions please feel free to call me or visit the TP web site I've been drafted to administer. http://terrapreta.bioenergylists.org/?q=node
It has been immensely gratifying to see all the major players join the mail list , Cornell folks, T. Beer of Kings Ford Charcoal (Clorox), Novozyne the M-Roots guys(fungus), chemical engineers, Dr. Danny Day of G. I. T. , Dr. Antal of U. of H., Virginia Tech folks and probably many others who's back round I don't know have joined.
This Earth Science Forum thread on these soils contains further links, and has been viewed by 40,000 self-selected folks. ( I post everything I find on Amazon Dark Soils, ADS here):
http://forums.hypography.com/earth-science/3451-terra-preta.html
Also Here is the Latest BIG Terra Preta Soil news;
ConocoPhillips Establishes $22.5 Million Pyrolysis Program at Iowa State 04/10/07
http://www.conocophillips.com/newsroom/news_releases/2007+News+Releases/041007.htm
Other Links;
University of Beyreuth TP Program, Germany http://terrapreta.bioenergylists.org/?q=taxonomy/term/118
__All the Bio-Char Companies and equipment manufactures I've found:
Carbon Diversion
http://www.carbondiversion.com/
Eprida: Sustainable Solutions for Global Concerns
http://www.eprida.com/home/index.php4
BEST Pyrolysis, Inc. | Slow Pyrolysis - Biomass - Clean Energy - Renewable Ene
http://www.bestenergies.com/companies/bestpyrolysis.html
Dynamotive Energy Systems | The Evolution of Energy
http://www.dynamotive.com/
Ensyn - Environmentally Friendly Energy and Chemicals
http://www.ensyn.com/who/ensyn.htm
Agri-Therm, developing bio oils from agricultural waste
http://www.agri-therm.com/
Advanced BioRefinery Inc.
http://www.advbiorefineryinc.ca/
Technology Review: Turning Slash into Cash
http://www.technologyreview.com/Energy/17298/
_The reason TP has elicited such interest on the Agricultural/horticultural side of it's benefits is this one static:
One gram of charcoal cooked to 650 C Has a surface area of 400 m2 (for soil microbes & fungus to live on), now for conversion fun:
One ton of charcoal has a surface area of 400,000 Acres!! which is equal to 625 square miles!! Rockingham Co. VA. , where I live, is only 851 Sq. miles
Now at a middle of the road application rate of 2 lbs/sq ft (which equals 1000 sqft/ton) or 43 tons/acre yields 26,000 Sq miles of surface area per Acre. VA is 39,594 Sq miles.
What this suggest to me is a potential of sequestering virgin forest amounts of carbon just in the soil alone, without counting the forest on top.
To take just one fairly representative example, in the classic Rothampstead experiments in England where arable land was allowed to revert to deciduous temperate woodland, soil organic carbon increased 300-400% from around 20 t/ha to 60-80 t/ha (or about 20-40 tons per acre) in less than a century (Jenkinson & Rayner 1977). The rapidity with which organic carbon can build up in soils is also indicated by examples of buried steppe soils formed during short-lived interstadial phases in Russia and Ukraine. Even though such warm, relatively moist phases usually lasted only a few hundred years, and started out from the skeletal loess desert/semi-desert soils of glacial conditions (with which they are inter-leaved), these buried steppe soils have all the rich organic content of a present-day chernozem soil that has had many thousands of years to build up its carbon (E. Zelikson, Russian Academy of Sciences, pers. comm., May 1994). http://www.esd.ornl.gov/projects/qen/carbon1.html
______________________
Erich J. Knight
What this suggest to me is a potential of sequestering virgin forest amounts of carbon just in the soil alone, without counting the forest on top.
Post a Comment
Links to this post:
Create a Link
<< Home